Since Yuri Gagarin’s first orbital flight in April 1961, mankind has been gaining experience of life in space. Nevertheless, only around 600 people have been into space so far. While the first space flights only lasted a few days, the Russian Mir space station in the mid-1980s and, since the turn of the millennium, the International Space Station (ISS) have made longer visits to space seem routine. It is foreseeable that more people will experience space in the future, not least because of private initiative. For example, multi-billionaire Elon Musk founded SpaceX in 2002 with the clear aim of one day establishing a Mars colony.
Future stays in a permanent lunar station or flights to Mars will pose completely new challenges to the fields of pharmacy and toxicology due to the conditions of space, in particular the low gravity, high radiation, large temperature differences and vacuum.
1 From Vostok to the ISS
As of March 26, 2024, there have only been 615 people who have ever been into space and orbited the Earth at least once. A further 71 people have reached an altitude of at least 80 km in suborbital flights, which is considered the limit to space. 24 astronauts have left the Earth’s gravitational field for the Moon, and 12 astronauts have actually set foot on our satellite (see Fig. 1 and Tab. 1).
Table 1. Space experience of mankind until March 26, 2024 [1].
Persons | Event |
---|---|
615 | Orbital flight |
247 | EVA (extravehicular activity) |
24 | Flight to the Moon |
12 | Walk on the Moon |
3 | Deep-space EVA |
Figure 1. Completely detached. Bruce McCandless was the first person to float completely free in space in 1984.
(Source grin.hq.nasa.gov at the Wayback Machine (archived 2001-02-23), Author: NASA)
At no time in the young history of space travel have more than 19 people been in space at the same time. Taken together, human beings have only accumulated a total of 187 years of spaceflight experience [1]. In fact, there are more Olympic champions, dollar billionaires, and Nobel Prize winners than people with space experience. The effects of low gravity or weightlessness (more precisely, microgravity) in orbit—on the moon the gravitational pull is 1/6 of the Earth’s gravity, on the ISS about 1/1000—is therefore an extremely rare experience for human beings.
Before manned space travel began, there was little knowledge of the effects of weightlessness on bodily functions—in particular circulation, consciousness, responsiveness and gastrointestinal activity. However, the effect of high g-forces during take-off and landing could be tested in a centrifuge. In 1950, the brothers Fritz and Heinz Haber developed the concept of parabolic flight [2]. In this way, one can experience a maximum of 30 seconds of weightlessness (see Fig. 2), enough time to practice simple body movements and putting on a spacesuit, but unsuitable for observing longer effects on the body. Ultimately, at the beginning of manned spaceflight, it was really only possible to draw on the experience of aviation medicine.
Figure 2. Parabolic flight—the only earthly path to short-term weightlessness.
(Source: Wessmann.clp)
Test pilots with at least 1,500 flight hours and an academic degree in a technical or scientific discipline qualified for the first astronaut programs in the USA. Pilots were also selected for the cosmonaut program in the Soviet Union. The final selection was then made through stress tests, which were more like torture than a job selection process.
Yuri Gagarin (1934–1968) was chosen from the final six cosmonaut candidates, who were generally believed to have the ability to survive the extreme physical stress of a first space flight. In addition to his KPDSU party membership, the Russian pilot and farmer’s son also had a smile that was to make him world-famous. On April 12, 1961, he set off on a 108-minute orbital flight with Vostok 1 and neither fainted nor went mad, but enjoyed a unique view of the Earth from a height of over 300 km. He was even able to eat a snack from a tube without any problems. Gagarin only had 247 flying hours when he joined the cosmonaut team. Luckily, no flying talent was required, as this space flight was fully automatic. After orbiting the Earth, he began his descent. However, the need to leave the capsule at an altitude of 7,000 m required a parachute jump, a fact that was concealed until the 1990s [3].
After Gagarin, the development accelerated, because ever since the Sputnik shock in 1957, when the Soviets launched the first satellite into space, the two superpowers had been engaged in a fierce struggle for supremacy in space, wanting to outdo each other at almost any cost. Just three weeks after Gagarin’s flight, the American Alan Shepard (1923–1998) managed a ballistic trajectory that lasted only 15 minutes but reached a maximum altitude of 187 km.
At the beginning of August 1961, Russian German Titov (1935–2000) was the first person to spend more than a whole day in space. At the age of 25, Titov was not only the youngest person to orbit the Earth to date, he also experienced the well-known space sickness (space adaptation syndrome SAS) with headaches and nausea for the first time after a few hours of weightlessness. The Soviets sent the first woman, Valentina Tereshkova (*1937), into orbit as early as 1963, but it was not until 1982 that the Russian Svetlana Savitskaya (*1948) became the second woman to fly into space.
In December 1964, the well-known biochemist Marcel Florkin (1900–1979) explained that anomalies in cell division meant that human beings could not survive for more than five days in zero gravity. Therefore, a successful journey to the moon would also be impossible, as the astronauts would arrive there dead [4]. However, in 1965, the Americans Gordon Cooper (1927–2004) and Charles Conrad (1930–1999) managed to spend more than a week in space with Gemini 5.
Just under five years later, Apollo 11 (see Fig. 3) with Neil Armstrong (1930–2012) and Buzz Aldrin (*1930) landed on the moon for the first time in July 1969. The first moon flight, with astronauts Frank Borman (1928–2023), William Anders (1933–2024), and Jim Lovell (*1928) orbiting the Earth’s satellite, had already taken place six months earlier with the Apollo 8 mission in December 1968. This also produced the famous image of the Earth rising above the lunar landscape.
Figure 3. Group photo with mankind. This picture from July 21, 1969, shows the lunar module with Armstrong and Aldrin above the surface of the moon. In the background is our home, Earth, with almost all the rest of humanity. Only Michael Collins is missing, as he took this photo from the command module.
(Source Author NASA / Apollo 11)
Shortly after the first landing on the moon, the legendary aerospace engineer and space architect Wernher von Braun (1912–1977) believed that a manned flight to Mars would be possible by 1982, and in an interview he said confidently, “I am firmly convinced that in the year 2000 we will have permanent research stations on the moon … in the year 2000, people who will be working in a moon station will say, I want to catch up with my family.” [5]. It turned out differently. For over half a century, since the Apollo 17 mission in December 1972, no human being has set foot on the moon.
While the American moon missions lasted a maximum of around two weeks, the stay in microgravity during the American Skylab missions in the early 1970s was extended to almost three months. During the Soviet missions on the Mir space station from 1986 onwards, the time spent in space was once again significantly increased. The Russian doctor and cosmonaut Valerie Polyakov (1942–2022) was in orbit for 437 days without interruption from January 1994 to March 1995, a record to this day. The US space shuttle missions were rather short, but made it possible to launch up to seven astronauts into space per flight. With the International Space Station (ISS), the number of astronauts and the time spent in space increased once again. The ISS has been permanently inhabited since the fall of 2000. As the space experience continues to grow, so do the requirements for the pharmaceutical care of the crews [6].
To this day, manned space travel remains a male domain, as only 77 of the 615 people who have ever been in orbit are female. Germany has sent 12 men into space to date, but no women. The private initiative “Die Astronautin” (The Female Astronaut), launched by aerospace engineer Claudia Kessler in 2016, did nothing to change this. Since November 2022, two Germans, former Eurofighter pilot Nicola Winter and medical doctor Amelie Schoenenwald, have been on ESA’s replacement astronaut team, both of whom are former participants in the aforementioned private initiative [7].
2 Effects of Weightlessness on Human Beings And Astropharmacy in Space
During the Mercury missions from 1961 to 1963, NASA constantly collected medical data from the astronauts during the space flights. Breathing rate, blood pressure, heart function, and body temperature were monitored with sensors. There was not yet an on-board pharmacy, but the spacesuits had four built-in injection syringes that could administer medication if necessary: pethidine (pain), metaraminol (shock), cyclizine (space sickness), and amphetamine (stimulant) [8].
With microgravity, coordinated behavior is more difficult because less force is required for body movement. Pressing buttons does not work, instead of scratching your head, you injure yourself with a violent blow because you use more energy than is necessary, or you bump into the spaceship wall. The eyes become the crucial organ for controlling movement. It takes about two days for astronauts to get used to this problem. With the heaviness of the body, with the loss of weight, there is also a loss of body awareness. Some astronauts feel as if they are just a spirit without a body. Parabolic flights, with their approximately 25 seconds of weightlessness, only prepare them to a limited extent for the permanent microgravity in orbit. The effects of an orbital flight on the human body are manifold (see Fig. 4).
Figure 4. Effects of microgravity on the human body.
[Silhouette: Source]
Around 60% of space travelers suffer from space sickness, the most exclusive motion sickness of all, which manifests itself in nausea and vomiting. These symptoms are treated with promethazine, usually for just one day. The motion sickness then disappears within about 48 hours. Hanging upside down on a pole for long periods of time or sleeping with your feet up are strategies to prevent space sickness, but with varying degrees of success.
Sleeping is more difficult, the noise level of the equipment is high, and the comforting cuddling with a blanket is not possible without gravity. This is why most space travelers take sleeping pills, usually zolpidem or melatonin.
As smells no longer reach the nose in zero gravity, food no longer seems to have as much flavor. This is why more seasoning is used.
Going to the toilet changes, and not just because a space toilet has to trap bodily excretions with a vacuum. Without gravity, urine does not simply collect at the bottom of the bladder, but sticks to the side of the bladder wall. The urge to urinate only becomes apparent when it is almost too late. Toilet visits should therefore be planned precisely according to the time of day.
Body fluids also shift from the legs to the head due to the lack of gravity. After just one hour, this is usually noticeable as a feeling of pressure in the head. Astronauts therefore have puffy faces.
Astronauts who have been on board the ISS for a longer time can be recognized by the fact that the calluses on their feet disappear, as they do not walk through the ISS but float without using their feet [9].
Because there is no natural gravitational load, muscle atrophy and around 1.5% bone loss per month occur. Two hours of daily strength training has become an effective measure against muscle atrophy. The decrease in bone density, on the other hand, cannot yet be stopped. A study of 17 astronauts showed that a six-month stay in orbit results in the same bone loss as 20 years of life on earth. Although bone loss is particularly severe in the first few months, there is no stabilization at all after six months. The arms are less affected than the legs. Astronauts who increase their training on the ISS are slightly less affected by bone loss than astronauts who train more before their stay in orbit than on the ISS [10].
The first pill taken in orbit was swallowed by Gordon Cooper in May 1964, when he took 5 mg of amphetamine sulfate shortly before re-entering the Earth’s atmosphere. Thirty-three hours of a tiring and lonely stay in his cramped Mercury capsule called for a stimulant. During the first, still short space flights, the stressful, lengthy launch preparations and the poorly air-conditioned spacesuits in the much too small spacecraft were the main source of physical strain.
The planned flight to the moon required longer space flights, even during the preparatory flights. A space pharmacy in the form of medical kits was used for the first time. Beforehand, a risk assessment had to be carried out to determine which illnesses were likely to occur during a two-week space flight, as the limited space restricted the choice of medication.
The first space pharmacy included ampicillin, cyclizine, amphetamine, secobarbital, pethidine, acetylsalicylic acid, and tetracycline. This selection was rather conservative, as NASA relied on the experience of military aviation. For sleeping pills, the well-known barbiturates were preferred to the then newly developed benzodiazepines such as diazepam (Valium®).
During the first Apollo flights, which were intended to prepare astronauts for the journey to the moon, NASA learned not only to perform the necessary rendezvous maneuvers (i.e., the targeted approach of two spacecrafts in space), but also to supplement the space pharmacy.
During the first flight in the Apollo capsule in October 1968, commander Walter Schirra (1923–2007) caught a cold and infected his colleagues Donn Eisele (1930–1987) and Walter Cunningham (1932–2023; see Fig. 5). Due to the lack of gravity, the nasal secretions could not drain downwards on their own and the astronauts had to constantly blow their noses. Although the technical tasks could be mastered well, the mood on board became worse and worse and culminated in a mutiny when the crew refused to put on their spacesuit helmets for re-entry into the Earth’s atmosphere. With their noses blocked, the astronauts feared major problems, including a burst eardrum. This paved the way for oxymetazoline, a rhinologic to reduce swelling of the nasal mucosa, which was first synthesized in 1961 and accompanied the Apollo 11 team on their flight to the moon in 1969.
Figure 5. Snuffly. The crew of Apollo 7 (Schirra, Eisele, Cunningham) were not only struggling with the new challenges of Apollo technology, but also with a cold. The sign says: Keep those cards and letters coming in folks.
( Source: ([1]) (JSC reference)
After the successful lunar flights, space travel focused on near-Earth orbit, but the stays in space were extended. As early as 1994, there were 200 different medicines and medical products on board the American space shuttles. The ISS is also equipped with resuscitation measures and operations, and the medical checklist even includes two pregnancy tests [11].
The use of medicines on the ISS hardly differs from the use of medicines during shorter space stays on space shuttle missions. Space sickness medication and sleeping pills are taken most frequently. In 54% of the cases, headaches and musculoskeletal pain have to be treated, mostly with ibuprofen and paracetamol. 55% of astronauts need medication for allergic reactions and nasal congestion.
The lack of gravity poses particular challenges for the dosage form of medicines, as simply taking a pill out of a tube carries the risk of it flying uncontrollably through the ISS and disappearing forever into a crack or an air filter. The solid dosage forms are therefore packaged in special large-volume blister packs. Microgravity is even more challenging for liquid drugs. Taking cough syrup from a dropper bottle becomes a magic trick without gravity. The application of nose and eye drops also becomes difficult if drops cannot fall downwards. Ampules for injections must be completely filled and without gas bubbles.
3 Radiation As a Danger to Human Beings And Medicines
3.1 Danger to Humans Beings
The significantly higher cosmic radiation poses a very great danger in space (see Tab. 2). The biological hazard results from the high-energy radiation, which has an ionizing effect, although the amount of energy as such is low. The amount of energy absorbed is called the equivalent dose. This used to be expressed in rem, but is now expressed in sieverts (Sv). 1 Sv corresponds to 100 rem. At a dose of 4 Sv, which is just 4 joules per kg of body weight, around 50% of those affected die within 30 days.
It has been shown that highly developed organisms react particularly sensitively to radiation. Doses as low as 0.5 Sv change the blood values in human beings, acute symptoms such as nausea and fatigue, hair loss, localized bleeding of the skin, and sterility appear from 1 Sv. From 6 Sv, the chance of survival is considered minimal (see Tab. 3).
Table 2. Additional annual cosmic radiation.
in mSv | |
---|---|
0 m | 0.3 |
2 km altitude | 0.6 |
10 km altitude (Airbus 380) | 40 |
18 km altitude (Concorde) | 90 |
300 km altitude (ISS) | 100 to 200 |
Living on Mars | 250 |
Expedition to Mars | 660 |
Table 3. Threshold limit values and typical dose values [38].
Effect | in mSv |
---|---|
Maximum dose from radiation on a flight from Frankfurt to Tokyo | 0.1 |
Average radiation | 2 |
Annual threshold limit value for employees in Germany | 20 |
Threshold limit value for damage to the unborn child | 100 |
Threshold limit value for the occupational lifetime dose of an employee | 400 |
Blood count changes/reddening of the skin | 500 |
Astronaut’s total career effective radiation dose (NASA) | 600 |
Threshold limit value for vomiting | 1,000 |
Threshold limit value for fatalities | 2,000 |
LD50 for minimum medical care | 4,000 |
LD50 for maximum medical care | 8,000 |
LD50 for trout | 15,000 |
LD50 for wasp | 1,000,000 |
The earlier the symptoms appear and the longer they last, the higher the radiation dose may be assumed to be. Recovery takes weeks or months. Low doses of radiation are associated with a higher long-term cancer rate. The risk of cancer at long-term doses of 100 to 200 mSv is epidemiologically proven. For doses below 100 mSv, the assessment is unclear. The annual tolerable dose for workers in nuclear power plants is 20 mSv. Despite years of research, the health consequences and risks for astronauts during long-term stays are still barely known [12].
While the annual radiation on the ground is around 0.3 mSv, it is 100 to 200 mSv on the ISS space station. For hypothetical life on a Mars station, it would be around 250 mSv. Due to the greater distance from the sun, only around 43% of the solar energy per surface area is likely to reach Mars compared to Earth. However, as there is no protective ozone layer in the Martian atmosphere, the intensity of UV radiation is considerably greater than on the Earth’s surface. In addition, sporadic solar flares cause an additional increase in radiation. NASA’s Curiosity probe measured the radiation exposure during its journey to Mars. A radiation level of 660 mSv was determined for a potential journey to Mars and back to Earth [13]. Possible eruptions on the sun could increase the values considerably.
For an entire working life, the legal maximum value in Germany on the ground is just 400 mSv. NASA sets the value for an astronaut’s lifetime at 600 mSv. This is not the only value that shows that astronauts are exposed to more dangers than in any other civilian profession. So far, 18 astronauts have died in four space accidents. This means that 3% of all space travelers have died in the course of their work!
3.2 Danger to Medicines
The higher radiation exposure in orbit is not only a danger for creatures. The medicine in the on-board pharmacy is also exposed to increased radiation, especially during long-term stays. Even within 10 days, significant differences were found in some cases between medicine on space shuttle flights and identical preparation sets on the ground. During 28 months on the ISS, six drugs in 15 samples had changed significantly, while only two of the products stored simultaneously on Earth had changed.
The number of drugs that lost their active pharmaceutical ingredient (API) content increased depending on the time in space. Some pills became crumbly and clumped together. Creams dried out or liquefied. The main components of ointments split. The high acceleration and deceleration during transportation are also not beneficial to the stability of medical substances [14]. So far, little is known about which products are created during decomposition and what measures are necessary to guarantee the stability of drugs.
As long as the ISS is in Earth orbit, this is not an issue, as there are eight or nine supply ships that deliver supplies to the ISS every year. A mission to Mars, on the other hand, would require about three years’ worth of supplies.
4 Investigation of Pharmacokinetics And Pharmacodynamics
In simple terms, pharmacokinetics examines what the body does with drugs. Pharmacodynamics, sees it from a different angle and investigates what drugs do to the body.
4.1 Pharmacokinetic Studies
Long-term pharmacological studies under microgravity conditions can currently only be carried out during space flights and on the International Space Station (ISS) (see Tab. 4). The cost of a ticket for a ten-day stay there is around 50 million euros. Thus, there are only three studies on pharmacokinetics that have been carried out under real space conditions. In one study, five astronauts were each given 325 mg of acetaminophen (paracetamol) on three different missions. The uptake of the active ingredient was increased in the first two days, while after four days the uptake decreased [15].
Table 4. Drugs that are frequently used in space [37].
Drug | Indication | Efficacy |
---|---|---|
Zaleplon, zolpidem, melatonin | Insomnia and sleep disorders | Moderately to very effective |
Ibuprofen, paracetamol | Pain | Moderately to very effective |
Glucocorticoids, fungicides, antihistamines | Skin rash | Moderately to very effective |
Promethazine | Space adaptation syndrome (SAS) | Very effective (prophylaxis) |
Another study investigated the pharmacokinetics of 0.4 mg scopolamine with 5 mg dextroamphetamine in 12 crew members. This showed a higher bioavailability than on Earth [16].
In the latest study, the pharmacokinetic properties of paracetamol were investigated in two dosage forms under long-term conditions. Five astronauts took 500 mg of active ingredient and were compared with a control group on earth. The bioavailability, half-life, and retention time of the drug increased in microgravity, while the absorption time decreased. Saliva samples were taken to determine the drug concentration, which might not reflect the situation in the blood [17].
4.2 Pharmacodynamic Studies
Pharmacodynamics in space, that is, the influence of active substances on the organism under microgravity conditions, are largely unexplored.
In order to at least partially investigate the consequences of weightlessness, the antiorthostatic bed-rest model (ABR) was developed. Healthy test subjects lie in a bed that is top-heavy and tilted downwards at an angle of 6 to 12 degrees. The body fluids shift from the legs towards the upper body and head, similar to a state of weightlessness.
During the experiments, all activities such as eating, drinking, and washing must be carried out lying down and at least one shoulder must remain in contact with the bed at all times. The test subjects are not allowed to receive visitors in their single room during the study and their correct posture is monitored by using cameras.
Long-term examinations also lead to bone and muscle loss, just like in space. The fact that an allowance of 16,000 euros is offered for three months’ participation in the trial shows just how difficult this experimental setup is for the test subjects [18]. Studies using the bed rest model are available for the active ingredients ibuprofen, paracetamol (painkillers), and promethazine (motion sickness). The release and absorption of ibuprofen are increased in simulated weightlessness, but no change in elimination or bioavailability was observed. A dose adjustment therefore does not appear to be necessary in space [19]. The pharmacokinetic properties of promethazine administered orally and intramuscularly were compared. Bioavailability is about 26% higher with oral administration. Presumably, there is a longer contact time between promethazine and the intestinal wall in simulated weightlessness [20].
4.3 Twin Study
The results to date militate against a special space dosage. However, even after more than 60 years of space research, the results of astropharmacology research are still rudimentary. The longest stays on the ISS usually last not much longer than six months. However, an expedition to Mars would take considerably longer. In order to clarify the question of how prolonged weightlessness and high radiation would change human beings, the most exclusive twin study in medical history to date was carried out.
The twins Mark and Scott Kelly (*1964) both made it into the NASA astronaut group of 1996 (see Fig. 6). While Mark Kelly spent just 59 days in orbit in four space flights, Scott Kelly was on the ISS for a whole year in 2015/2016. During this year, he received an additional radiation dose of 146 mSv. This corresponds to the natural dose of 50 years on Earth.
The results indicate that Scott aged faster in orbit than his brother Mark, who remained on Earth. Scott’s chromosome ends, the telomeres, were shortened, the combination of speed and accuracy in solving standardized tasks was reduced, inflammation parameters were increased, the carotid artery was thickened, and the quality of the eyes was reduced [21].
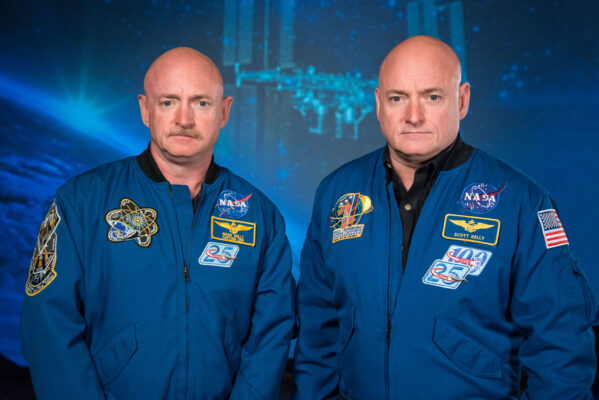
Expedition 45/46 Commander, Astronaut Scott Kelly along with his brother, former Astronaut Mark Kelly speak to news media outlets about Scott Kelly’s 1-year mission aboard the International Space Station. Photo Date: January 19, 2015. Location: Building 2. Photographer: Robert Markowitz
Figure 6. The Nasa twin study: Mark (right) and Scott (left) Kelly are the only pair of twins ever to have been in space, Scott almost a year longer than his brother Mark.
(Autor: NASA/Robert Markowitz)
Another problem with a Mars mission is that the radiation exposure of solar flares is even higher than on the near-Earth ISS. Special protection will therefore be necessary in future Mars spacecrafts; the age of Mars astronauts will have to be higher, to minimize the risk of cancer later in life In addition, NASA has already stipulated that one or even two doctors must be on board for a flight to Mars.
5 Astrotoxicology
Toxicology is already a constant companion of pharmacology and pharmacy on Earth. The same applies to the infinite expanses of space. In contrast to toxicology on Earth, astrotoxicology must take into account the special features of the extreme conditions in terms of gravity, radiation, temperature, and pressure in space, especially in the highly complex artificial system of spacecraft and, last but not least, on other celestial bodies. Astrotoxicology is therefore an interdisciplinary science that combines elements of medicine, chemistry, astronomy, physics, biology, and toxicology (see Fig. 7).
Figure 7. Astrotoxicology as a multidisciplinary science [39].
So far, it has hardly been mentioned in textbooks on toxicology or poisons [22]; however, astrotoxicology is primarily concerned with:
- the special toxicological conditions in spaceships
- the dangers of increased radiation in space for human beings and matter
- the special toxicological conditions caused by the extreme conditions in space
- the influence of low gravity on the toxicological properties in the human body
The world experienced a dramatic and yet weird introduction to astrotoxicology with the flyby of Haley’s comet in 1910. Shortly before this, hydrogen cyanide had been detected spectroscopically in the comet’s tail. Scientific discussions and a mass press fixed on sensationalism fueled wild speculation with increasingly absurd reports.
The hydrogen cyanide from the tail was supposed to soak the atmosphere and wipe out life on Earth. Some media, such as the Los Angeles Examiner, took it with a sense of humour and wrote, among other things, “Has the comet cyanized you yet? … free gas bath for all mankind … many already feel a harsh cyanic taste on their tongues … cheers, hilarity to be expected.” Resourceful profiteers took the opportunity to sell gas masks and anti-comet pills [23]. When the comet was closest to Earth on May 20, 1910, the end of the world did not come, because the concentration of hydrogen cyanide in the tail was far too low to have a harmful effect, and the Earth’s atmosphere is also a natural barrier for extraterrestrial substances.
5.1 Air
The beginning of manned spaceflight then marked the serious beginning of astrotoxicology, and the earliest toxicological risk concerned the possible contamination of the air for breathing in a space capsule. After an explosion in the command module during the Apollo 13 mission, the crew escaped into the lunar module, which was only intended for two people. The carbon dioxide content then rose to 2% due to the air breathed by three astronauts. The normal value for a spacecraft is 0.4%, which is ten times higher than in the Earth’s atmosphere.
In 1975, when an Apollo capsule returned from a rendezvous with a Russian Soyuz capsule, there were problems during the landing process. As a result, nitrogen dioxide from the fuel entered the Apollo capsule, causing one of the astronauts to faint. At times there were around 700 ppm of nitrous oxide in the space capsule, 700 times more than the threshold limit value (TLV). After returning to Earth, the crew remained in hospital for two weeks under medical observation [24].
There were repeated leaks in the cooling system on the Russian Mir space station. This caused ethylene glycol to escape, which irritated the crew’s mucous membranes and, above all, their eyes.
Fires are always a problem in space travel, also because of the carbon monoxide they produce. After a cellulose filter caught fire, cosmonauts complained of headaches and nausea. Analysis revealed a CO value of 400 ppm. Later analyses showed that as much as 40% of the haemoglobin in the blood was blocked by the CO.
Since 1992, NASA has established spacecraft maximum allowable concentrations (SMACs) for 56 hazardous substances in the atmosphere of their spacecrafts. The SMACs apply to the breathable air. For each substance, values were defined for one hour, one day, one week, one month, six months, and 1,000 days. The threshold limit values are naturally set higher for short periods of time than for longer ones. 1,000 days is the period that a Mars mission could last (see Tab. 5) [25].
Table 5. Comparison of some maximum permissible concentrations on the ISS (SMAC compared to maximum workplace concentrations MAK in Germany) [25], [40]; SMAC in ppm.
Compound | 1 hour | 1 day | 7 days | 30 days | 180 days | 1,000 days | MAK |
---|---|---|---|---|---|---|---|
Ethanol | 5,000 | 5,000 | 1,000 | 1,000 | 1,000 | 1,000 | 200 |
Hydrogen | 4,100 | 4,100 | 4,100 | 4,100 | 4,100 | ||
Freon 22 (refrigerant) | 1,000 | 1,000 | 1,000 | 1,000 | 1,000 | 500 | |
Carbon monoxide | 425 | 100 | 55 | 15 | 15 | 15 | 28 |
Xylene | 50 | 17 | 17 | 17 | 8.5 | 1.5 | 50 |
Ammonia | 30 | 20 | 3 | 3 | 3 | 3 | 20 |
Ethylene glycol | 25 | 25 | 5 | 5 | 5 | 10 | |
Hydrocyanic acid | 8 | 4 | 1 | 1 | 1 | 1.9 | |
Formaldehyde | 0.8 | 0.5 | 0.1 | 0.1 | 0.1 | 0.3 | |
Mercury | 0.01 | 0.002 | 0.001 | 0.001 | 0.001 | 0.005 |
5.2 Water
There is a water-recycling system on the ISS that recovers drinking water from urine; some of this water is used for oxygen production. Long-term stays in space, especially future trips to Mars, will certainly require a recycling system for water. Guideline values for the recycled water—spacecraft water exposure guidelines (SWEGs)—have now been defined for 30 chemical compounds. NASA has been working on water recycling systems since 1986 and is now able to turn over 90% of the wastewater on the ISS back into clean water.
Urine is first treated with the oxidizing agent chromium trioxide and an inorganic acid such as sulfuric acid. This decomposes microbes and chemical substances. The pre-treated liquid is then fed into the urine processor assembly (UPA). Water and volatile substances then evaporate at low temperatures and low pressure.
Under Earth conditions, the water vapor rises and residues remain. Without gravity, this separation is not possible, which is why the evaporation process takes place in a rotating drum on the ISS. The resulting artificial gravity collects the heavy substances on the inside of the drum. It is reminiscent of the accelerated settling of a fine precipitate in a centrifuge in the practical exercise for undergraduate students in chemistry. The water vapor remains in the middle and is pumped to a multi-filter system. There, large organic compounds are adsorbed, while inorganic impurities are removed by ion exchangers. Finally, substances with a small molecular weight are removed by catalytic oxidation. What remains is pure water (see Fig. 8).
Figure 8. Water recovery system on the ISS.
According to [26], NASA; Silhouette: freepik.com, author: flaticon
Water treatment on the ISS is a success story. However, there was also a surprising problem with the initial application. On Earth, the urine processor had been tested with 90 mg of calcium per liter, but due to natural bone degradation under microgravity conditions, the ISS crew’s urine contained around 230 mg of calcium per liter. As a result, so much calcium sulfate was precipitated in the distiller that the entire system calcified and failed. This was remedied by reducing the water recovery rate to 75%. Changing the chemical mixture increased the rate back to 85% [26].
The SWEGs, that is, the threshold limit values for the treated water, are staggered in 1- and 10-day values, for a short-term higher amount of pollutants that can still be tolerated by the crew. The values are set lower for 100 and 1,000 days. Water that smells or tastes bad over a long period of time could lead to the crew consuming too little water in the long term, which could jeopardize the entire mission on future trips to Mars [27]. The threshold limit values of the German Drinking Water Ordinance are much stricter, as Table 6 shows.
Table 6. Comparison of some spacecraft water exposure guidelines (SWEG) on the ISS with German threshold limit values of the Drinking Water Ordinance [27], [41]; SWEG in mg/L.
Compound | 1 day | 10 days | 100 days | 1,000 days | German threshold limit value for drinking water |
---|---|---|---|---|---|
Ethylen glycol | 270 | 140 | 20 | 4 | |
Dichloromethane | 40 | 40 | 40 | 15 | |
Benzene | 21 | 2 | 0.7 | 0.07 | 0.001 |
Manganese | 14 | 5.4 | 1.8 | 0.3 | 0.05 |
Antimony | 5 | 1 | 1 | 1 | 0.005 |
Nickel | 1.7 | 1.7 | 1.7 | 0.3 | 0.02 |
Cadmium | 1.6 | 0.7 | 0.6 | 0.022 | 0.003 |
Lead | 0.009 | 0.009 | 0.01 |
Water remains a scarce and valuable commodity in spaceships, so personal hygiene is carried out using damp cloths. A shower was only used on the Skylab in the early 1970s, but has not proved practical. Clothing is worn for as long as possible and collected in a space transporter after use. Ultimately, this transporter burns up together with the waste on re-entry into the earth’s atmosphere.
There are no plans for a laundry on the ISS. The aforementioned Scott Kelly jumped straight into his swimming pool in full gear when he returned home after his one-year stay on the ISS and never wanted to take water in such large quantities for granted again [28].
6 The Toxicology of Space
So far, we have only dealt with the toxicology on board the spaceship, a man-made artificial virtually terrestrial oasis in the middle of a hostile universe. Large temperature differences, different gravity, vacuum, but also high pressure, radiation, and extreme dryness can enable the formation of unusual toxicological substances.
6.1 Moon Dust
Moon dust, regolith, was in fact the first extraterrestrial substance that human beings encountered during space travel. Due to the lack of an atmosphere, the moon is always affected by meteorite impacts. This produces very fine dust. 10 to 20% is fine dust with a particle diameter of less than 20 µm, up to 2% of the regolith is smaller than 3 µm. This lunar regolith consists of aluminosilicates, ferromagnesium silicates, feldspar, or olivine—compounds that are basically also known on Earth. Analysis of the rock samples brought back by the Apollo missions revealed around 45% SiO2, 15% Al2O3, 12% CaO, 14% FeO, 9% MgO, and 1% Na2O.
However, the lunar dust is in an ultravacuum and is exposed to high UV and X-ray radiation and very high temperature differences during the day. While dust and sand grains on Earth are ground round by weathering over time, the lunar dust grains on the atmosphere-free lunar surface remain sharp-edged and become electrostatically charged. It is assumed that the surface of the lunar dust is activated in this way.
The moon travelers on the Apollo missions noticed that the moon dust stuck to their spacesuits over time and covered all the equipment in the lunar module. In addition, the smell of gunpowder spread throughout the spaceship. Harrison Schmitt (*1935), the 12th and last person to walk on the moon, spoke of a lunar hay fever after returning to Earth, which was accompanied by sneezing, a scratchy throat, and watery eyes. Once on Earth, the rock lost its characteristic smell.
The moon travelers were unable to carry out more detailed toxicological tests on site. To further look into it, fresh lava ash was examined as imitation moon rock in 2018. When freshly ground up, the grains are as sharp-edged as moon dust. In fact, tests with this imitation resulted in significant cell damage. At a concentration of 20 mg per 3.8 cm2, only 10% of the cells survived. The small size of the dust particles increased the potential reaction surface, and penetration into the cells was also possible [29]. This potential danger would be a further challenge when operating a future moon base.
6.2 Perchlorate Salt
In addition to increased radiation, other unusual chemical risks lurk on an interplanetary journey. The Wet Chemistry Laboratory of the Phoenix Mars Lander found a remarkably high concentration of 0.5–1% perchlorate salt on the surface of Mars [30]. This is three to four orders of magnitude more than on the Earth’s surface. Presumably, the perchlorate was produced by the high UV radiation on Mars. As perchlorates are oxidizing agents, they also make bacterial life directly on the surface unlikely. Model experiments on Earth showed that perchlorates accelerated the cell death of bacteria by a factor of 10 [31].
An exact investigation of the surface of Mars is limited by the fact that it has never been possible to return material from Mars to Earth with a probe. On the one hand, perchlorates could be an easily accessible source of oxygen, but they are also a source of danger for future astronauts [32]. The intake of perchlorates leads to a reversible inhibition of iodine uptake by the thyroid gland. Iodine deficiency can lead to a lack of the thyroid hormone thyroxine in human beings. A Mars struma could therefore be a possible Mars-specific disease in the distant future.
6.3 Many Unknown Questions
But what about the influence of low gravity on the toxicological properties of chemical substances in the human body? Should the lethal doses LD50 be different in space? There are no LD50 values determined in space, and the general study situation under real space conditions is poor. On May 8, 2015, ESA astronaut Alexander Gerst visited his old university in Hamburg, Germany, where he had completed his doctorate in geophysics in 2010, to give a lecture in the Audimax. The author of this article was also present at a press conference held beforehand (see Fig. 9). When asked whether he had noticed a change in his sensitivity to medication or alcohol in microgravity, Gerst replied: “I didn’t notice any change in my body’s reaction to substances on the ISS. Incidentally, alcohol is banned on the ISS, but I don’t know what the Russians do in their part of the station.”
Figure 9. Astroalex. On May 8, 2015, the author (left) met ESA astronaut Alexander Gerst at a press conference at the University of Hamburg.
[Own picture]
7 Prospects
The special features of space complement the classical natural sciences of physics, chemistry, and biology with the sub-fields of astrophysics, astrochemistry, and astrobiology. It is becoming apparent that something similar will happen for pharmacy and toxicology. The University of Nottingham, UK, for example, now has its own working group that conducts research solely in the field of astropharmacy. Its aims include the development of microsensors for measuring physiological function in space, non-invasive control of bone decalcification, elucidation of the higher virulence of bacteria, new methods for simulating microgravity, or quality assurance of drugs in space [33].
So far, the contribution of pharmacists to the health of astronauts has been rather small [34], but it is foreseeable that the rapid development of manned space flight will lead to a significant increase in this area [35]. The same is likely to apply to toxicologists. Finally, let’s take a look into the future of space travel.
First Landing on Mars
The former ESA astronaut Ulrich Walter ventured a prediction for the exact date of the very first landing of human beings on Mars: If we take off from Earth on April 11, 2048, Earth and Mars will be in such a favorable alignment that the flight would only take 113 days. Astronauts could then set foot on the surface of Mars for the first time on Sunday, August 2, 2048 [36]. If it were possible to combat natural bone resorption under low gravity, a major challenge in astropharmacy, a permanent stay on Mars would also be possible. Perhaps then, with 1/3 of the Earth’s gravity, corks will fly high at a champagne reception on August 2, 2098 to celebrate the possible 50th anniversary of the first landing on Mars.
Does champagne get you drunk faster on Mars than on Earth? Astrotoxicology will have answered this question by then.
Summary
The history of spaceflight has been shorter than an average human lifespan. Since 1961, around 600 human beings have been in orbit. The extreme conditions of space add a new astronomical dimension to all the sciences known to us on Earth and require many protections and adaptations for a permanent space stay. It is foreseeable that the progress of manned space spaceflight, especially future new moon missions or even flight to Mars, will bring great impulses to the field of astropharmacy and astrotoxicology in the next decades.
Acknowledgments
My thanks go to Astrid Menzel, who drew my attention to the press conference with ESA astronaut Alexander Gerst in May 2015 and who translated this present text. This experience encouraged me to think about pharmacy and toxicology under space conditions. I would also like to thank Patricia Pesel, who, as a qualified mineralogist, gave me some tips about moon rocks, and Klaus Strey for reviewing the German manuscript.
References
[1] Joachim Wilhelm Josef Becker, Heinz Hermann Janssen, Space Facts, December 15, 2023. (accessed May 27, 2024)
[2] F. Haber, H. Haber, Possible methods of producing the gravity-free state for medical research, J. Aviat. Med. 1950, 21(5), 395-400.
[3] S. Walker, Ins All, Hoffmann und Campe, Hamburg, Germany, 2022. ISBN: 978-3-455-01088-6
[4] SCIENTIST VOICES MOON TRIP DOUBT; Belgian Says Weightless Effects Could Be Fatal, New York Times 24. December 1964. (accessed May 27, 2024)
[5] RÜCKSTURZ ZUR ERDE, Der Spiegel, February 7, 1971. (accessed May 27, 2024)
[6] C. Staiger, Pharm. Ztg. 2009, 154, 14-22.
[7] Die Astronautin GmbH, Prinzhöfte, Germany. (accessed May 27, 2024)
[8] Christiane Staiger, 50 Jahre Apollo 11, Pharm. Ztg. 2019.
[9] S. Cristoforetti, Die lange Reise – Tagebuch einer Astronautin, Penguin, München, Germany, 2019. ISBN: 978-3328601036
[10] Leigh Gabel, Anna- Maria Liphardt, Paul A Hulme, Martina Heer, Sara R Zwart, Jean D Sibonga, Scott M Smith, Steven K Boyd, Pre-flight exercise and bone metabolism predict unloading-induced bone loss due to spaceflight, Br. J. Sports Med. 2022, 56, 196-203. https://doi.org/10.1136/bjsports-2020-103602
[11] Emily S. Nelson, Arnon Chait, Glenn Research Center, Cleveland, OH, USA, Portable Diagnostics Technology Assessment for Space Missions, NASA/TM–2010-215845/PART2, February 2010.
[12] J. C. Chancellor, R. S. Blue; K. A. Cengel, S. M. Aunon-Chancellor, K. H. Rubins, H. G. Katzgraber, A. R. Kennedy, Limitations in predicting the space radiation health risk for exploration astronauts, npj Microgravity 2018, 4, 8. https://doi.org/10.1038/s41526-018-0043-2
[13] C. Zeitlin et al., Measurements of Energetic Particle Radiation in Transit to Mars on the Mars Science Laboratory, Science 2013, 340(6136), 1080-1084. https://doi.org/10.1126/science.1235989
[14] B. Du, V. R. Daniels, Z. Vaksman, J. L. Boyd, C. Crady, L. Putcha, Evaluation of physical and chemical changes in pharmaceuticals flown on space missions, AAPS J. 2011, 13, 299-308. https://doi.org/10.1208/s12248-011-9270-0
[15] “Inflight pharmacokinetics of acetamin in saliva”, N. M. Cintron, L. Putcha in Results of Life Science DSOs Conducted Aboard the Space Shuttle 1981-1986, NASA, pp. 19-23.
[16] “Inflight salivary pharmacokinetics of scopolamine and dextroamphetamine”, N. M. Cintron, L. Putcha, J. M. Vanderploeg in Results of Life Science DSOs Conducted Aboard the Space Shuttle 1981-1986, NASA, pp. 25-29.
[17] I. V. Kovachevich, S. N. Kondratenko, A. K. Starodubtsev, L. G. Repenkova, Pharmacokinetics of acetaminophen administered in tablets and capsules under long-term space flight conditions, Pharm. Chem. J. 2009, 43, 130-133. https://doi.org/10.1007/s11094-009-0255-6
[18] Maylis Jean-Preau, Payés 16 000 euros pour rester allongés pendant soixante jours, Le Parisien, December 13, 2017.
[19] N. Idkaidek, T. Arafat, Effect of microgravity on the pharmacokinetics of Ibuprofen in humans, J. Clin. Pharmacol. 2011, 51, 1685-1689. https://doi.org/10.1177/0091270010388652
[20] P. Gandia, S. Saivin, A. P. Le-Traon, A. Guell, G. Houin, Influence of simulated weightlessness on the intramuscular and oral pharmacokinetics of promethazine in 12 human volunteers, J. Clin. Pharmacol. 2006, 46, 1008-1016. https://doi.org/10.1177/0091270006291032
[21] F. E. Garrett-Bakelman et al.,The NASA Twins Study: A multidimensional analysis of a year-long human spaceflight, Science 2019, 364(6436). https://doi.org/10.1126/science.aau8650
[22] K. Strey, Die Welt der Gifte, 3rd ed., Lehmanns Media, Berlin, Germany, 2021. ISBN: 978-3-96543-210-9
[23] C. Sagan, Unser Kosmos, Droemer Knauer, München, 1982.
[24] N. Khan-Mayberry, J. T. James, R. Tyl, C-W. Lam, Space Toxicology: Protecting Human Health During Space Operations, Int. J. Toxicol. 2011, 30, 3-18. https://doi.org/10.1177/1091581810386389
[25] Spacecraft Maximum Allowable Concentrations for Airborne Contaminants, NASA April 2020.
[26] L. Carter, Überlebensnotwendige Technik im All, Spektrum 2017.
[27] SPACECRAFT WATER EXPOSURE GUIDELINES (SWEGs), NASA, July 2017.
[28] S. Kelly, Endurance – Mein Jahr im Weltall, Bertelsmann, München, 2018.
[29] R. Caston, K. Luc, D. Hendrix, J. A. Hurowitz, B. Demple, Assessing Toxicity and Nuclear and Mitochondrial DNA Damage Caused by Exposure of Mammalian Cells to Lunar Regolith Simulants, GeoHealth 2018, 2, 139-148. https://agupubs.onlinelibrary.wiley.com/doi/10.1002/2017GH000125
[30] M. H. Hecht et al., Detection of Perchlorate and the Soluble Chemistry of Martian Soil at the Phoenix Lander Site, Science 2009, 5936, 64-67. https://doi.org/10.1126/science.1172466
[31] J. Wadsworth, C. S. Cockell, Perchlorates on Mars enhance the bacteriocidal effects of UV light, Sci. Rep. 2017, 7, 4662. https://doi.org/10.1038/s41598-017-04910-3
[32] A. F. Davila, D. Willson, J, D. Coates, C. P. McKay, Int. J. Astrobiol. 2013, 12, 321-325.
[33] School of Pharmacy: Astropharmacy and Astromedicine, Nottingham University, UK. (accessed May 27, 2024)
[34] I. Sawyers, C. Anderson, M. J. Boyd, V. Hessel, V. Wotring, P. M. Williams, L. S. Toh, Astropharmacy: Pushing the boundaries of the pharmacists’ role for sustainable space exploration, Res. Social. Adm. Pharm. 2022, 18(9), 3612-3621. https://doi.org/10.1016/j.sapharm.2022.02.002
[35] S. Aziz, M. A. Raza, M. Noreen, M. Z. Iqbal, S. M. Raza, Astropharmacy: Roles for the Pharmacist in Space, Innov. Pharm. 2022, 13(3). https://doi.org/10.24926/iip.v13i3.4956
[36] U. Walter, Im schwarzen Loch ist der Teufel los, Komplett-Media, 2016.
[37] J. Kast, L. Laqua, R. Möcker, H. Derendorf, Schwerelos, Schwerelos – Arzneimitteltherapie im Weltraum, Dtsch. Apoth. Ztg. 2017, 24, 50-54.
[38] Grenzwerte im Strahlenschutz, Bundesamt für Strahlenschutz (BfS), Salzgitter, Germany (accessed May 27, 2024)
[39] K. Strey, Astrotoxicology, Preprints 2019, 2019060120. https://doi.org/10.20944/preprints201906.0120.v2
[40] MAK- und BAT-Werte-Liste 2023, Deutsche Forschungsgemeinschaft, Bonn, Germany.
[41] Bundesgesetzblatt Teil I, Zweite Verordnung zur Novellierung der Trinkwasserverordnung, Nr. 159, Bonn, Germany, 20. Juni 2023.
The Author
Dr. Karsten Strey, Germany
Karsten Strey received his doctorate from the University of Hamburg, Germany, in 1996 on theoretical organic chemistry. His knowledge of toxicology and pharmacy, which he acquired as a medical journalist and pharmaceutical consultant, inspired him to write the book “Die Welt der Gifte” (The World of Poisons), which is now in its third edition. He has been a regular contributor to the magazine Chemie in unserer Zeit for several years.
The article has been published in German as:
- Ziemlich abgehoben – Astropharmazie und -toxikologie in der Raumfahrt,
Karsten Strey,
Chem. unserer Zeit 2024, 58, 2–13.
https://doi.org/10.1002/ciuz.202300015
and was translated by Astrid Menzel.