Polymeric materials, commonly referred to as “plastics”, suffer from a bad public image these days: The connotation that determines our relation to plastics is clearly not as positive as it used to be. An Australian study finds that the public perception focuses on “plastic waste” and the environmental contamination that is caused by it, while there also is an association with the convenience (not quality) provided by plastic-wrapped products [1].
1 Plastic: A Synonym for Progress, Independence, and Overcoming Poverty
Nowadays plastic has an image problem, but its story started quite differently. Even if today we have trouble viewing plastics, i.e., polymers, neutrally or even positively—there were and are good sides to the “plastic frenzy”. The term “plastic” had positive connotations for many years and decades, synonymous with progress, independence, and even overcoming poverty.
Whether we discuss building materials, utilitarian objects, or toys—mankind has always used materials to influence its environment and to make life easier or simply more enjoyable: Wooden beams in houses, insulation material made of straw and clay, piano keys made of ivory, dolls made of porcelain, clothes made of wool or plant fibers, closed with buttons made of metal or horn.
Plastic has replaced many of these biological or mineral materials, turning items that were once luxuries reserved for a few into everyday objects that can be affordably produced and purchased by (nearly) everyone. We take it for granted that our children can choose from—sometimes too many—toys and games. However, this has only been made possible by the development of plastics that can be produced cheaply and molded easily, that have a low weight, and that feature material properties that can be controlled and tuned in a facile way.
Let us take one of the simplest children’s toys: the ball. Balls made of leather, filled with animal hair or feathers, were already known in Europe in pre-Christian times. A few centuries later came the first air-filled balls, made of animal bladders. These balls were more or less round—but neither very elastic nor very stable.
2 Ambiguity: Ecological and Social Burden
Charles Goodyear described vulcanization in 1839, which suddenly made it possible to create elastic balls that had a higher bounce and were much easier to kick. However, this was based on natural rubber, a natural product that was either produced in Asia in huge rubber plantations with high fungicide use and poor working conditions—or came from “natural” sources in South America, which were also with overexploitation and clear-cutting. Here we see one of the biggest drawbacks of natural materials: They are accessible and purchasable only by a selected few, usually in the so-called first world, and often create an ecological and social burden for many.
Fritz Hofmann applied for a patent on synthetic rubber in 1909 [2,3]. Just three years later, the first fully synthetic tires were produced, so there was no longer any need to rely on natural rubber. The adaptability of the material not only allowed the production of tires but also permits a steadily increasing number of diverse applications.
But like so much of the history of plastics, this development is marked by ambiguity: The patent Hofmann applied for, like so many of the chemical-technical developments of the time, must be placed in the context of the First World War: The German Reich could no longer obtain enough natural rubber on the world market, and the German industry, which was directly linked to many of the atrocities of the war, found a solution.
Today, balls in all colors and sizes can be purchased cheaply—and some of them have become high-tech products, where in addition to the main components of the ball, polyurethane layers are also bonded.
3 Plasticizers and Other Additives: The Good and the Bad
Starting from a simple kid’s toy, we already see part of the problem. In our childhood, most balls were just flexible rubber materials, but nowadays, even these supposedly simple objects are made from composite materials to enable material engineering and property tuning. And the same is true for polymers: We probably all learned at school that polymers are composed of chains of varying lengths and can be grouped into three classes:
- Thermoplastic materials, which have very few cross-links between the chains and are therefore readily meltable,
- Elastomers, which have more crosslinks that allow a flexible return to the individual chain positions and confer high elasticity to the materials, and
- Duromers, which are highly connected between the chains, resulting in resistant, strong materials.
3.1 Additives
This classification suggests that the different polymer classes literally consist of polymer chains. However, if we have a look at the commonly used polymer PVC, or polyvinylchloride, we see that the reality is very far from this simple picture. PVC indeed consists of long chains of connected vinylchloride monomers (chloroethane), but the pure polymer is a white and brittle powder that is rarely ever used. Only very few niche applications use pure (or additive-free) PVC at all for highly specific material coatings in lab settings.
Most of the PVC products in daily life rely on some type of additive to tune the properties of the pure polymer: Tubings, for example, regularly contain filler materials (e.g., fibers or particles) that confer stability and resistance. Cables used in settings where they have to be resistant to, e.g., heat or sparks, often contain compounds that confer thermal inertness such as phenols or benzophenones. To make the polymers interact with light, or simply tune their appearance, pigments are frequently incorporated.
At this point, it is worth mentioning that while most polymers are rather non-toxic and inert materials, additives do add significant risks — even if their concentration in the polymer is generally small! To highlight this, let us look at plasticizers: They are often molecules with long side chains, used to soften the materials. This class of additives has been much discussed after the turn of the millennium because of their hormone-like effects on organisms. After several studies could prove the impact on young children and marine organisms, several plasticizers were banned in children’s objects within the European Union [4].
3.2 Leakage of Plasticizers
Let’s add a short story to illustrate how impactful the leakage of plasticizers is: In 2010, Juliane Simmchen did a research stay at the Catalonian Anti Doping Laboratory, more specifically, in the group of Jordi Segura, who was, at that time, developing a strategy to detect blood doping by evaluating the presence of plasticizers in an individual’s blood. Own-blood doping is a technique in which athletes extract a part of their blood, and re-inject the red blood cells later, increasing the body’s capacity to transport oxygen, which gives a competitive advantage in endurance sports. This means that even though only about 10 % of the blood had actually been stored in a plasticized PVC bag, the amount of plasticizers that leaked into the blood (typically stored in refrigerated conditions) is large enough and reproducible be to used in crime detection [5].
In the meantime, awareness about the effects of plasticizers has grown so much that many people have moved away from plastic food containers and store their food in glass containers, often with bamboo lids [6].
The amount of plastic we produce every year is increasing [7], and most of the plastic is used as packaging. Of course, we have to discuss the reasons for this, whether this is necessary, and whether there might be better alternatives.
4 Great Advantage: The Low Weight of Polymers
Why do we fill beverages into PET bottles, when glass or ceramic bottles are also available? In general, glass bottles have a longer life and can be recycled repeatedly without losing quality. Plastic bottles, on the other hand, have a shorter life span and can only be recycled a limited number of times before the material degrades. So why are the PET bottle or container still a mainstay in our everyday life?
The manufacturing process of glass bottles requires a significant amount of energy, and they are heavier, resulting in higher emissions during transport. In contrast, plastic bottles have a smaller manufacturing and transportation footprint. The impact of this lower footprint is enormous: The weight of a glass bottle can be up to 40 times higher than that of a comparable PET bottle.
This is what makes calculating and comparing the actual footprint of both materials so difficult—because it is not just the recycling rate that plays a role, but also the transport distance: A jam imported from across the Atlantic Ocean in a glass jar has a much higher environmental footprint than a locally produced jam in a PET jar.
The low weight of polymers is also an advantage in other applications, particularly in transport: In cars, not only are tires and seats made of plastics, but also parts of the bodywork, dashboard components, and engine. It is estimated that plastics account for about 50 % of the volume of a car on average—but only about 10 % of the total weight of a car. Replacing plastic would, therefore, increase the weight of the car, requiring more energy (from fossil or sustainable sources) to propel it forward. Similar calculations can be made for other forms of transportation from airplanes to e-scooters. In all cases, the low weight of the plastics used is a significant advantage. Cutting down long transport routes for produce and products could have a high impact on sustainable and conscious consumerism.
5 Plastic Waste: The Downside of Durability and Resistance
It is clearly more sustainable to produce and consume all of our daily needs locally, but our common habits and the convenience of having most products and types of produce available at all times will be hard to change. The price we pay for this convenience are about 150 million tons of plastic waste in the oceans [8], with roughly 8 million tons added each year [9].
Looking at the origin of this plastic waste, it becomes apparent that countries with long coastlines are more important contributors to oceanic contamination, but also that recycling and waste management strategies in Europe have improved our plastic (waste) footprint, at least when we only consider what ends up in the ocean. Global plastic production and single-use products are unfortunately still on the rise, and worrying about environmental issues is often still a privilege. Many regions of the world have not yet reached the necessary level of wealth and education to dedicate their energy to environmental protection. On the other side, richer and more developed countries often have lifestyles that promote fast food and consumerism and thereby create more waste.
We have all seen impactful photos of turtles with plastic straws, dolphins suffering in fishing nets, and deformed turtles that have grown inside of a six-pack holder. We are aware that the impact of plastic waste on the environment is drastic. Part of this problem originates from the long lifetimes of plastic—their durability and resistance are among the most unique and desired features of different polymeric materials. The other side of the medal is, however, that whatever enters the environment does not degrade and remains present for centuries.
However, this visible part of plastic waste is not the only problem, even if the polymer does not disintegrate or decompose, shear, radiation, and mechanical stress contribute to breaking them into smaller pieces, i.e., nano- and microplastics. Because of their inert nature, these particles pose serious analytical limitations to detectability and have gone unnoticed for long. As of now, they have been found on all continents, including in arctic ice and even within the human body.
However, the actual danger and toxicity of microplastics for humans is still a matter of ongoing research. While certain aspects have been confirmed, for example, that microplastics enhance the accumulation of contaminants such as heavy metals, and that small organisms often starve due to accumulation of microplastic in their digestive tracks, a large part of its impact is yet unexplored or requires further studies.
It is very clear that microplastics are really difficult to remove once they have been released into any environment. Traditional water treatment plants that are frequently based on filtering or flocking techniques remove almost none of the microplastics. Therefore, innovative solutions like the silanization of microplastics to cause precipitation, the use of magnetic nanoparticles for mechanical removal, or even our microscope scale collection by tiny micromotors [10], now even with the ability to photocatalytically degrade the microplastic, are usually well-received because of the lack of a real, practical solution. Sometimes it seems that a ban of single-use plastic is really the only solution.
6 Materials and Protective Gear: Plastics Keep Us Safe
At the same time, plastics, i.e., polymers, are “safe” in many ways: They are (at least generally) not electrically conductive, and have, thus, accompanied the construction of our electrified and networked society, keeping us safe in the most trivial of ways: as insulators that protect us from electric shocks. The mechanical properties of plastics can be adapted and controlled in just as many ways: We can use them not only to produce elastic balls with great bounce, but also engineer plastic for high impact resistance, which is important for safety equipment like helmets or airbags. Also, the good chemical and thermal resistance of plastics make them ideal for use in products exposed to harsh chemicals or environments.
Another point we often take for granted: Plastics save lives. The medical world uses plastic devices, from syringes to prosthetic limbs. On an individual and very personal level, we also use plastics to be safe: During sexual intercourse as condoms, in the laboratory as disposable gloves, or during a global pandemic in disposable masks.
In the field of medicine in particular, we can see how much we in the first world take plastics for granted: Sterile and hygienic medical items, from gloves to syringes to catheters, are still not available to everyone in this world as “unlimited” single-use materials. Banning single-use plastic would primarily burden people in less developed regions, who often already lack vital single-use items and cannot afford to switch to sustainable products that will inevitably be more expensive and less readily available.
7 Recycling: Still Difficult
Still, protective gear (similar to convenience products) often just lands in water streams and landfills and contributes to the overall waste amount. Additionally, the protective gear is frequently contaminated or potentially contaminated, and, therefore, not recyclable, which brings us back to a lot of waste. Globally, the amount of waste produced annually is still rising. The Heinrich Böll Foundation reported that only about 9 % of all plastics ever produced have actually been recycled, which includes a certain part of incinerations [11].
Recycling can take place at three different levels: as materials, as raw or primary products, and energetically. Given that plastics are made from fossil fuels, ending up in landfills or the ocean is not even making use of the energetic content of plastics. One major problem is that the quality of recycled plastic is frequently much lower compared with the starting material. Even for products where recycling is frequently promised, it is practically implemented only for a very limited percentage of plastic waste. The simplest example is probably plastic bottles made from polyethylene terephthalate (PET).
Located between Germany (which has had a return, or “Pfand” system for more than 20 years now) and Scotland (where currently the return system is not yet in place), Juliane Simmchen clearly sees the value of it and the impact it has on cleanliness in the city. PET is a material for which the impact on material quality after recycling is rather low. The bottles are sorted by color, shredded and washed, dried, and subsequently melted and shaped into pellets, that can be used to produce new items. My favorite example is the EMECO111 chair, which used the material of 111 PET bottles in its fabrication process [12].
Transferring this process to a wider range of materials is often difficult, not only because of the use of additives and composites that can hardly be recycled. There is a lot of applied and basic research going on in this regard. Investigating catalysts that facilitate the controlled breakdown of plastics into their monomers is very important for the development of new strategies for the circular economy—and to make them more profitable.
It is clearly a disaster that making polymeric materials out of fossil fuels is so much cheaper than recycling waste products. Fixing this is an important task for chemistry and chemical engineering that requires massive investments. To address this issue, many countries and companies are implementing measures to increase plastic recycling, such as improving the recycling infrastructure, designing products to be recyclable, and promoting consumer education and behavior change. In the end, even consistently burning plastic is better than burning raw oil while landfilling plastic, so at least, the plastic can be considered a useful intermediate.
In general, every material used should be considered a valuable resource. This does not only encompass the conscious and respectful handling of commodity products made from plastic, but also keeping a focus on developing new production processes with a decreased environmental footprint, e.g., by tapping into new and more sustainable starting materials. In addition, the degradation of polymeric materials needs to be investigated further to enhance our options to induce a controlled breakdown into non-toxic degradation products. Furthermore, new plastic materials and material classes have to be developed.
Looking at green technologies, one has to note that these are relying on finely tuned polymeric materials: from energy harvesting, e.g., the lightweight construction of rotor blades in wind parks, to energy storage, e.g., polymeric membranes in new battery technologies, to benign new materials for specialized medical applications (from wearable sensors to smart sutures). However, maintaining continuity in people’s behavior seems crucial to ensure the avoidance of single-use polymeric materials wherever possible, proper recycling when needed, and responsible disposal if recycling is not feasible. Therein, the commitment of each individual is as important, as is having sustainable regulations in place.
Acknowledgment
J. S. acknowledges a Freigeist grant (No. 91619) from the Volkswagen Foundation and a Fulbright Cottrell Award, which partially supported the microplasic study.
References
[1] L. S. Dilkes-Hoffman, S. Pratt, B. Laycock, P. Ashworth, P. A. Lant, Public attitudes towards plastics, Resour. Conserv. Recycl. 2019, 147, 227–235. https://doi.org/10.1016/j.resconrec.2019.05.005
[2] Fritz Hofmann, Patent Nr. 250690, Patent – Verfahren zur Herstellung von künstlichem Kautschuk, 1909.
[3] Jens Soentgen, Ein deutscher Stoff: Synthesekautschuk in Deutschland, 1909–2009 in Lost in Things – Fragen an die Welt des Materiellen (Eds. Philipp W. Stockhammer, Hans Peter Hahn), Band 12, Tübinger Archäologische Taschenbücher, Germany, 2015. ISBN 978-3-8309-3175-1
[4] K. Christen, Controversial EU phthalate ban here to stay?, Environ. Sci. Technol. 2000, 34. https://doi.org/10.1021/es0034803
[5] J. Simmchen, R. Ventura, J. Segura, Progress in the removal of di-[2-ethylhexyl]-phthalate as plasticizer in blood bags, Transfus. Med. Rev. 2012, 26, 27–37. https://doi.org/10.1016/j.tmrv.2011.06.001
[6] H. C. Erythropel, M. Maric, J. A. Nicell, R. L. Leask, V. Yargeau, Leaching of the plasticizer di(2-ethylhexyl)phthalate (DEHP) from plastic containers and the question of human exposure, Appl. Microbiol. Biotechnol. 2014, 98, 9967–9981. https://doi.org/10.1007/s00253-014-6183-8
[7] R. Geyer, J. R. Jambeck, K. L. Law, Production, use, and fate of all plastics ever made, Sci. Adv. 2017, 3, e1700782. https://doi.org/10.1126/sciadv.1700782
[8] E. Kosior, I. Crescenzi in Plastic waste and recycling (Ed. Trevor Letcher), Elsevier, Amsterdam, Netherlands, 2020, 415–446. ISBN: 9780128178805
[9] Y. Geng, J. Sarkis, R. Bleischwitz, How to globalize the circular economy, Nature 2019, 565, 153–155. https://doi.org/10.1038/d41586-019-00017-z
[10] Linlin Wang, Andrea Kaeppler, Dieter Fischer, Juliane Simmchen, Photocatalytic TiO2 Micromotors for Removal of Microplastics and Suspended Matter, ACS Appl. Mater. Interfaces 2019, 11(36), 32937–32944. https://doi.org/10.1021/acsami.9b06128
[11] Heinrich Böll Foundation: Plastik Atlas 2019; Facts and figures about the world of synthetic polymers
[12] Emeco 111: Navy Chair (accessed July 10, 2023)
Authors
Prof. Dr. Franziska Lissel, Leibniz-Institut für Polymerforschung Dresden e. V., Germany
Dr. Juliane Simmchen, Freigeist Physical Chemistry, TU Dresden, Germany
Also of Interest
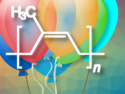
The discovery of vulcanization—a happy accident or the result of hard work?
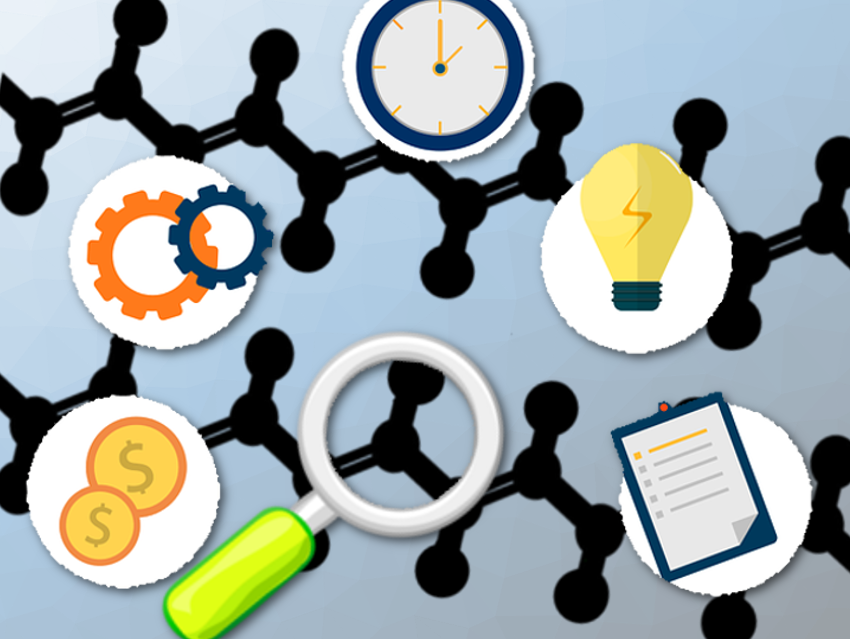
Board members and editors of a science journal have analyzed their future visions
For me as a polymer chemist from industry, it is very gratifying to read again positive things about plastics. Of course, in the future, plastics must be handled even more responsibly and in a way that conserves resources. After all, it is thoughtless to “dispose” of waste – not just plastic waste – in the environment, because nature gives it all back to us one way or another.
In this aspect, the article unfortunately adopts the exaggerations of the opponents of plastics. According to them, plastics would remain in the environment for hundreds of years. But there are no serious sources for this! Not only since the discovery of the glacier corpse Ötzi is it known that organic material is durable under suitable conditions for a very long time. But does this fit to all plastic garbage?
There are alarmingly high figures circulating about the amount of plastic waste in the world’s oceans. What is the scientific quality of the published figures on which the authors rely? How could these figures be substantiated! Two years ago, the GEOMAR Institute started a search project because random samples found only about 10% of the stated quantities. Had the majority already been mined, sunk or never arrived there in the first place? But even 10% is too much garbage in the sea.
How the authors come to the statement that wastewater treatment plants retain almost no microplastics is incomprehensible. According to the state of the art, sewage treatment plants retain about 95% of microplastics. But if sewage sludge is then spread on the fields, the microplastics are only displaced. It would be better to incinerate this sewage sludge.
It is gratifying that the authors break with the taboo of incinerating plastic waste. They see incineration as a viable alternative to dumping plastic waste in the environment. The polymer chemist also thinks that if the plastic has been used well and sensibly, it can be additionally recycled for energy afterwards. Try that with a brick!
Basically: thanks for this article.