Our teeth are there to help us smile our way through life. But what happens when they fail us? Bacterial activity, brute force, or gum inflammation are just some reasons why we may need to fill cavities, repair fractures, restore crowns, or replace an entire tooth with an artificial one.
Fortunately, we now have access to a variety of materials to regain good dentition, often for as long as an original tooth would last. From gold to ceramics, mercury alloys to composites, cements, and ceramics, restoration materials have to fulfill a number of criteria. They must be strong and durable, but also easy to work with, to seal cavities or create a proper artificial crown. Implants must be biocompatible, and adhesive systems need to be able to attach the restoratives to the dentin and enamel of the remaining teeth.
How do these materials work? Let’s take a closer look at the chemistry behind fixing our smiles.
1 Restoring the Crown: Dental Fillings and Inlays
1.1 Strength, Durability, Biocompatibility: The “True” Gold Standard
Gold is malleable to very fine structures, extremely durable, and perfectly biocompatible. It has been used in restoration since ancient times and is still in use today. Around 630 BC, the Etruscans, a wealthy ancient culture that lived in Italy before the Roman Empire, were the first to make gold wire dental bridges to fill the gap between two teeth when one was lost. This was done by attaching a hollow gold tooth to a thin band of gold anchored to the healthy teeth.
The gold not only had the function of replacing teeth, but it also signified that its wearer was wealthy. Gold in the mouth was considered attractive, a notion that still holds true for many around the world today.
Today, dental gold is used as an alloy to increase its strength, since pure gold is too soft and can be deformed by chewing. High gold alloys contain at least 60% gold and 10–20 % palladium-group metals. Low-percentage gold alloys only contain less than 12 % gold, the rest is silver (30–60 %), palladium-group metals, and copper and zinc.
With proper care, gold crowns and inlays can last for decades. Gold does not corrode, crack or chip off when used in dental restorations. Dental gold has thus set a standard that other restorative materials are yet to meet.
1.2 Filled with Promise: Amalgam
However, gold is expensive, and it cannot simply be filled into a cavity. Instead, healthy tooth material has to be ground away to prepare the surface for mounting inlays or crowns. In contrast, dental amalgam, a metal alloy invented in the mid-1800s, only hardens after it has been filled into the cavity. To this day, it is still used as a simple and inexpensive way to seal cavities caused by decay in many countries.
Dental amalgam is the combination of one part liquid mercury with one part metallic alloy containing silver and tin. When these parts are mixed and compacted, a setting reaction occurs, causing atomic silver and tin to migrate into the bulk of the mercury, creating crystalline phases. Once set, the amalgam forms a tough, durable solid that can last for up to 15 years with proper handling and good oral hygiene.
Figure 1. Schematic representation of an amalgam filling.
Nevertheless, the use of amalgam is declining for several reasons. One is a lingering concern over the use of toxic mercury, even though many studies have shown that the amount of mercury released from the filling is no greater than the amount of mercury the body is exposed to naturally in the environment [1].
Another reason is that its metallic finish is considered unattractive, with white dental fillers being preferred. Finally, amalgam fillings need mechanical anchoring to remain in place. This means that the dentist will grind out perfectly healthy tooth material to prepare the undercuts for anchoring the alloy, a procedure that modern dentistry would like to move away from.
“Today, dentists can operate with greater precision,” says Professor Anne Katrin Lührs, a specialist in restorative dentistry at the Medical University of Hannover, Germany. Advances in composite technology and, in particular, adhesive systems have made it possible to seal cavities and fissures of almost any shape and size over the long term, she says.
1.3 Applied in Layers: Dental Composites
Composite resins are also known as tooth-colored fillings. They provide strength and durability by combining a synthetic resin, usually an acrylic polymer, with a filler material, usually silica-based. Composite resins can be color-matched to a patient’s natural tooth color, making them a popular choice for cosmetic dental procedures.
1.3.1 Where Chemistry Meets Physics: Modern Dental Adhesives
Although the color and strength of composites match those of the tooth substance, other properties do not. The natural tooth structure is hydrophilic, while the composite resin is hydrophobic. The dental adhesive must be designed to bond both structures.
Dentin, the hard inner substance of the tooth, is composed of collagen (20 % by weight) uniformly impregnated with tiny crystals of hydroxyapatite. In contrast, enamel, which is the tooth’s outer protective layer, contains only about 0.6 % calcified organic matter, the remainder being thicker (about 50 nm x 50 nm) hydroxyapatite crystals.
Figure 2. Schematic representation of the anatomy of a tooth.
Modern adhesive systems exploit the weakness of these hydroxyapatite crystals to acids. When exposed to acids, the crystals dissolve, releasing calcium and phosphate ions. This leads to the demineralization of dentin and enamel, leaving a porous collagen layer in the dentin with micrometer-sized pits and grooves. An adhesive resin can physically anchor in this layer to form a hybrid layer, a mechanism called micromechanical retention.
If phosphate groups are present in the adhesive resin, for example, by using a phosphate-containing monomer (see Fig. 3), the phosphate can bind ionically to the calcium ions of the hydroxyapatite. This bonding mechanism is known in dentistry as chemical adhesion. Modern dental adhesive systems always combine micromechanical retention and chemical adhesion.
The adhesive itself is an acrylic acid resin. It is applied as a monomer solution that polymerizes when activated by light in the presence of an initiator. Functional monomers contain phosphate groups for adhesion, but can also contain hydroxy and acid groups for further wetting, etching, and bonding purposes.
Figure 3. Glycerol phosphate dimethacrylate (GPDM) was the first functional monomer and is still used in some popular dental adhesive products.
Etching is a crucial step in the adhesive process. It occurs in part through the action of acidic functional monomers (the “self-etch” strategy), but it is significantly enhanced when the surface is treated with phosphoric acid before the monomer solution is applied. This technique, known as “etch-and-rinse”, deeply demineralizes the dentin for strong micromechanical retention. However, excessive demineralization can also destabilize the dentin structure, risking hydrophilic degradation from within the tooth.
When acids extract calcium ions from the hydroxyapatite, these calcium ions can also be involved in a novel, but very useful adhesion mechanism. In the early 1980s, scientists at Kuraray in Japan discovered that 10-methacryloyloxydecyl dihydrogen phosphate (10-MDP, see Fig. 4) provided an extraordinary adhesion strength. It soon turned out that this was because 10-MDP assembled in stable nanolayers held together by the extracted calcium ions (see Fig. 5).
Figure 4. 10-Methacryloyloxydecyl dihydrogen phosphate (10-MDP) is an adhesive monomer.
Since then, 10-MDP has found an entry in most adhesive systems as a functional monomer. It provides the methacrylate group for polymerization, a hydrophobic spacer for nanolayer assembly, and the anionic phosphate group calcium bonding. This three-dimensional layered structure adds strength and resistance to acids and water, although 10-MDP still has some hydrolytic weakness due to the two ester bonds.
Figure 5. 10-MDP assembled in stable nanolayers held together by the extracted calcium ions; calcium in green.
Since the 1950s, dentists have lived through eight generations of adhesives [2], each intended to improve chemical or physical bonding to both tooth hard substances, surface preparation, or handling. The most recent development is the “universal adhesives,” which are adhesive formulations that allow the clinician to decide on a case-by-case basis whether to use the etch-and-rinse strategy or the milder self-etch technique, and even which restorative material will work best.
1.3.2 Light Curing: Composite Resins
Once the adhesive system has been applied to the prepared tooth surface, dried, and cured, the composite resin is added to complete the restoration. Composite resins combine a continuous organic matrix – an acrylic polymer – with dispersed filler particles made of ceramics, mainly silica. The acrylic polymer will form a continuous matrix when cured, and the filler provides stability and form.
Figure 6. Schematic representation of a composite filling.
However, this organic–inorganic combination has a problem: the silica may break away from the matrix during curing and over time. In the early 1960s, Rafael Bowen, a dentist working for the American Dental Association Foundation, solved this problem by adding a silane coupling agent, which originally was tris(2-methoxyethoxy)(vinyl)silane [3]. Since then, organosilanes, such as 3-(trimethoxysilyl)propyl methacrylate (see Fig. 7) have found their way into all dental formulations where silica-based materials need to be bonded to organic materials.
Figure 7. 3-(trimethoxysilyl)propyl methacrylate
Bowen’s other invention was the use of bisphenol-A glycidyl methacrylate (bis-GMA, see Fig. 8) as a monomer that polymerizes to form a stable, efficiently cross-linked polymer matrix. Refinements have since been made to the quality and quantity of filler, the curing method, fluidity adjustments, and the inclusion of stabilizers and pigments.
Figure 8. Bisphenol-A glycidyl methacrylate (bis-GMA).
The resins are cured by activating camphor quinone as the photoinitiator using light at a wavelength of 468 nm. This form of curing has been gradually replacing the earlier two-component systems, which cure by a chemical reaction.
1.4 All Set: Cements
Apart from amalgam and composite resins, a third class of materials is available for filling and sealing purposes: cements. Dental cements vary enormously in their compositions and prescribed uses, but most have a glass-like or metal oxide component, such as fluoro-aluminosilicate glass or zinc oxide, and are set either by an acid–base reaction (with polyacrylic acid, for example) or chelation (with eugenol, for example) [3].
Dental cements do not require additional adhesive systems: they are themselves dental adhesives. This is because their polyacid component both slightly etches the tooth’s surface and forms anionic bonds for chemical retention. These properties make them easy to apply, which has many advantages.
For example, in children’s dental care, glass ionomer cements (the combination of fluoroaluminosilicate powder and polyacrylic acid) might be preferable to composite resins, as Anne-Katrin Lührs explains: “The use of dental composites means a rather elaborate treatment process. The tooth needs to be dry, then you apply the adhesive, which needs to be spread evenly over the area in question before being cured with light. Only then is the composite material applied in layers, with each individual layer being cured by light before the next is applied. A bulk fill composite might be an alternative, as it can be applied in one layer, but still the application of the adhesive and the composite remains a technique sensitive step. This procedure is relatively complex and might not be tolerated by some of our younger patients. Glass ionomer cement, on the other hand, can basically be applied in one layer and used as an alternative restorative material, depending on the clinical situation.”
On their own, though, cements are more prone to wear and tear than other filling materials and can only be used temporarily. Despite this, their variability in composition, ranging from metal oxides to composite-like polymer resins, means they can be used in a variety of bonding applications. Cements can be used to fix metallic and ceramic preformed components such as inlays, crowns, retainers, bridges, and dentures to the tooth, a process known as luting. They are also used to seal root canals and protect the dental pulp.
1.5 Most Pleasant: Ceramics
Dental ceramics are strong, rigid, inert, thermally and electrically insulating, and have the white-translucent appearance of natural teeth. For all these reasons, they are used for indirect esthetic restorations. Indirect means that they are first molded and then cemented to the tooth stump. Their disadvantage is that they are more brittle than dental polymers and alloys, because their strong, directional bonding structure makes them very resistant to compression but more vulnerable to failure under tensile stress.
Figure 9. Schematic representation of a ceramic filling.
Ceramics are a mixture of oxides, silicates, borates, and fluorides, mostly formed from light metals, with both glass and crystalline components. They are made by the thermal fusion of powders in the presence of additives such as pigments, and the sintered components are held together by ionic and covalent bonds. As fusion is also possible for metal oxides, metals or metal alloys forming such oxides often form the core of a crown that is coated with a fused ceramic layer.
2 A Question of Biocompatibility: Implants
Titanium implants have been state-of-the-art in dentistry since the pioneering work of Swedish orthopedic physician Per-Ingvar Brånemark. In the 1950s, Brånemark discovered that titanium prostheses integrate with bone tissue without being rejected or causing inflammation. This biotolerant integration, called osseointegration, has led to the extraordinary success of titanium and titanium alloys for dental implants to the present day.
However, ceramics can also be osseointegrated, and yttria-doped zirconia forms an exceptionally strong and fatigue-resistant ceramic, dubbed “ceramic steel” [4]. In addition, zirconia has a dental color, whereas titanium can glance unfavorably in a dark gray color through the gum. Zirconia implants have been introduced in the 1990s, and have come increasingly into focus in recent years when preparation techniques have advanced even further.
In addition, bacteria can form biofilms on the micro-etched titanium surface. Microstructured surfaces facilitate the growth of fibroblasts, thereby promoting osseointegration. However, they also promote bacterial adhesion, which can develop into a harmful inflammatory condition called peri-implantitis, leading to implant failure. This occurs on titanium surfaces, which can be corroded by the action of bacteria [6], but apparently less so on zirconia [7].
Are zirconia implants then better than titanium ones? Not per se, says Philipp-Cornelius Pott, senior physician and specialist in dental prosthodontics at Hannover Medical School. After all, zirconia is a ceramic material, and ceramics are brittle, which means they are generally less resistant to tensile stress than metals. Pott argues that the studies currently available on modern ceramic implants do not yet allow evidence-based conclusions to be drawn about their long-term clinical behavior.
This means that, at least for the near future, esthetic zirconia will not simply overtake functional and long-established titanium. Current research is focusing on the surface properties of titanium and titanium alloys to further improve their mechanical properties and at the same time prevent bacterial colonization [8].
References
[1] Monika Rathore, Archana Singh, Vandana A. Pant, The Dental Amalgam Toxicity Fear: A Myth or Actuality, Toxicol Int. 2012, 19(2), 81–88. https://doi.org/10.4103/0971-6580.97191
[2] Bart van Meerbeek, Kumiko Yoshihara, Kirsten van Landuyt, Yasuhiro Yoshida, Marleen Peumans, From Buonocore’s pioneering acid-etch technique to self-adhering restoratives. A status perspective of rapidly advancing dental adhesive technology, J. Adhes. Dent. 2020, 22, 7–34. https://doi.org/10.3290/j.jad.a43994
[3] Stephen C. Bayne, Beginnings of the dental composite revolution, J. Am. Dent. Assoc. 2013, 144(8). https://doi.org/10.14219/jada.archive.2013.0205
[4] Robert G. Craig, Dieter Welker, Josef Rothaut, Klaus Georg Krumbholz, Klaus-Peter Stefan, Klaus Dermann, Hans-Joachim Rehberg, Gertraute Franz, Klaus Martin Lehmann, Matthias Borchert, Dental Materials, in: Ullmann’s Encyclopedia of Industrial Chemistry, Wiley-VCH, Weinheim, Germany, 2006. https://doi.org/10.1002/14356007.a08_251.pub2
[5] Alvaro Della Bona, Oscar E. Pecho, Rodrigo Alessandretti, Zirconia as a dental biomaterial, Materials 2015, 8, 4978-4991. https://doi.org/10.3390/ma8084978
[6] R. Raphael C. Costa, Victoria L. Abdo, Patrícia H. C. Mendes, Isabella Mota-Veloso, Martinna Bertolini, Mathew T. Mathew, Valentim A. R. Barão, João Gabriel S. Souza, Microbial Corrosion in Titanium-Based Dental Implants: How Tiny Bacteria Can Create a Big Problem?, J. Bio. Tribo. Corros. 2021. https://doi.org/10.1007/s40735-021-00575-8
[7] Stefan Roehling, Monika Astasov-Frauenhoffer, Irmgard Hauser-Gerspach, Olivier Braissant, Henriette Woelfler, Tuomas Waltimo, Heinz Kniha, Michael Gahlert, In Vitro Biofilm Formation on Titanium and Zirconia Implant Surfaces, J. Periodontol. 2017, 88(3), 298–307. https://doi.org/10.1902/jop.2016.160245
[8] Jafar Hasan, Richard Bright, Andrew Hayles, Dennis Palms, Peter Zilm, Dan Barker, Krasimir Vasilev, Preventing Peri-implantitis: The Quest for a Next Generation of Titanium Dental Implants, ACS Biomaterials Science & Engineering 2022, 8(11), 4697–4737. https://doi.org/10.1021/acsbiomaterials.2c00540
Also of Interest
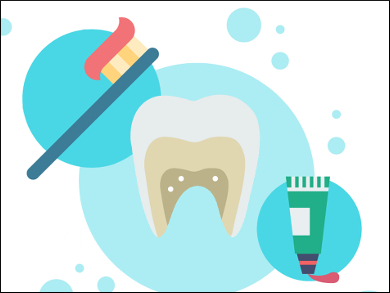
Why fluoride is good for your teeth
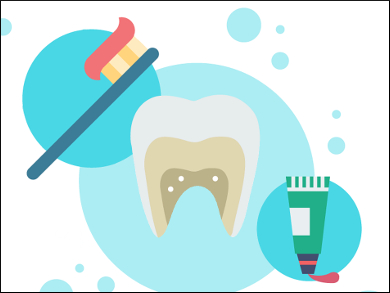
How to brush your teeth and how toothpaste works
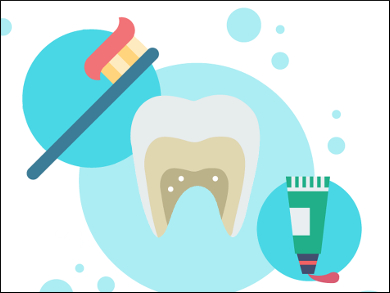
What teeth are made of and the causes of tooth decay
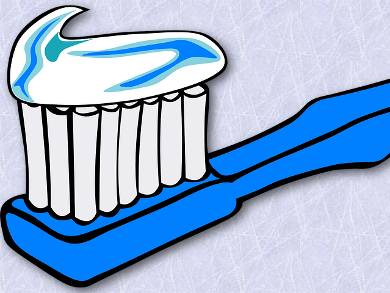
Looking at the latest trends and what really works